The actin cytoskeleton is an intricate polymer system that can be assembled into many different structures, ranging from stable stress fibers that locally support cell adhesion, to the extensive and dynamic branched network at the leading edge that drives cell migration. However, a question that keeps intriguing the field is how this plethora of structures, each with their own particular turnover rates and physical properties, can be assembled in the same cytosol from the same basic building blocks, i.e. actin filaments. In other words, what determines the fate of an actin filament?
In the Jansen lab, we are using a combination of genetics, biomimetic systems, cell imaging, multi-color in vitro TIRF microscopy and single molecule techniques to investigate how two important, but heavily understudied families of actin-binding proteins, the Tropomyosins and the Coronins, play a role as regulatory hubs that generate different actin filament populations that are optimally suited to mediate intracellular trafficking, cell migration, cytokinesis or cell adhesion.
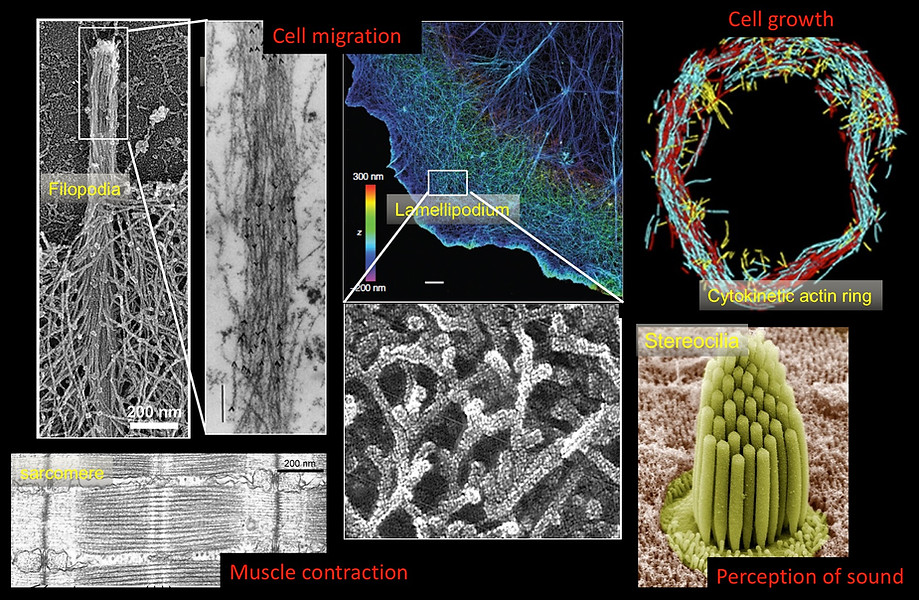
The actin cytoskeleton is an intricate polymer system that can be assembled into many different structures, ranging from stable stress fibers that locally support cell adhesion, to the extensive and dynamic branched network at the leading edge that drives cell migration. However, a question that keeps intriguing the field is how this plethora of structures, each with their own particular turnover rates and physical properties, can be assembled in the same cytosol from the same basic building blocks, i.e. actin filaments. In other words, what determines the fate of an actin filament?
In the Jansen lab, we are using a combination of genetics, biomimetic systems, cell imaging, multi-color in vitro TIRF microscopy and single molecule techniques to investigate how two important, but heavily understudied families of actin-binding proteins, the Tropomyosins and the Coronins, play a role as regulatory hubs that generate different actin filament populations that are optimally suited to mediate intracellular trafficking, cell migration, cytokinesis or cell adhesion.
greatly hampered by their overlap in genetic sequence, which has made it hard to discern one isoform from another, or target a specific isoform for depletion in cells and in vivo model systems.
To get a better understanding of the actin-TPM barcoding system, we are leveraging the expertise of the lab in single-molecule multicolor TIRF microscopy to characterize the different F-actin populations formed by the biologically most relevant mammalian TPMs (Shown in figure above). Using this approach, we showed that protection of actin filaments from disassembly varies widely depending on the TPM isoform decorating the filament (Shown in figure below), and thus TPM decoration specifies actin populations with different disassembly rates, and may be an important mechanism to tune actin network turnover in cells.

A. Left, schematic representation of F-actin disassembly by Coronin 1B, Cofilin 1, and AIP1. Right, snapshot of preassembled actin filaments (in cyan) being disassembled by Coronin 1B, Cofilin 1 (in yellow), and AIP1. B. Same as A but with TMP added to the reaction mixture. C. Treadmilling reconstitution assay in which filaments are actively being assembled at one end by mDia1 (formin) while simultaneously being disassembled by Coronin 1B, Cofilin 1 (in yellow), and AIP1 at the other end. Decoration with different TPM isoforms results in filaments displaying different turnover behavior.
Tropomyosin
​
Tropomyosins (TPMs) are coiled-coil proteins that can assemble into long polymers that wrap around actin filaments. Over the years, the extensive family of TPMs has been shown to regulate the interaction of filamentous actin with many other actin-binding proteins in cells. As such, decoration with different TPM isoforms is hypothesized to generate actin filaments with specific physical properties and binding capacities, and thus indicate different filament populations, much like a biological “barcode”. In line with the great diversity in actin structures, eukaryotes have 4 TPM genes that can be alternatively spliced into more than 40 TPM isoforms. However, delineating the precise characteristics that each TPM endows actin filaments with has been
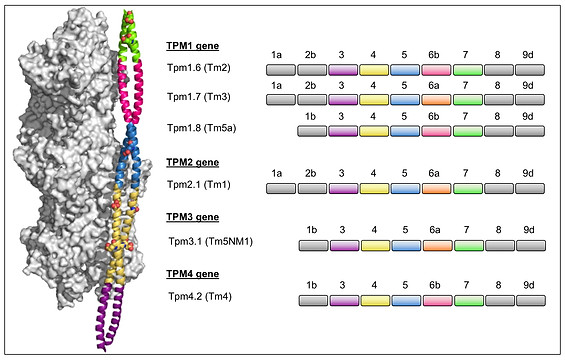